New Research Shows How Nerve Impulses Travel, May Offer Insights to Effects of MS Demyelination
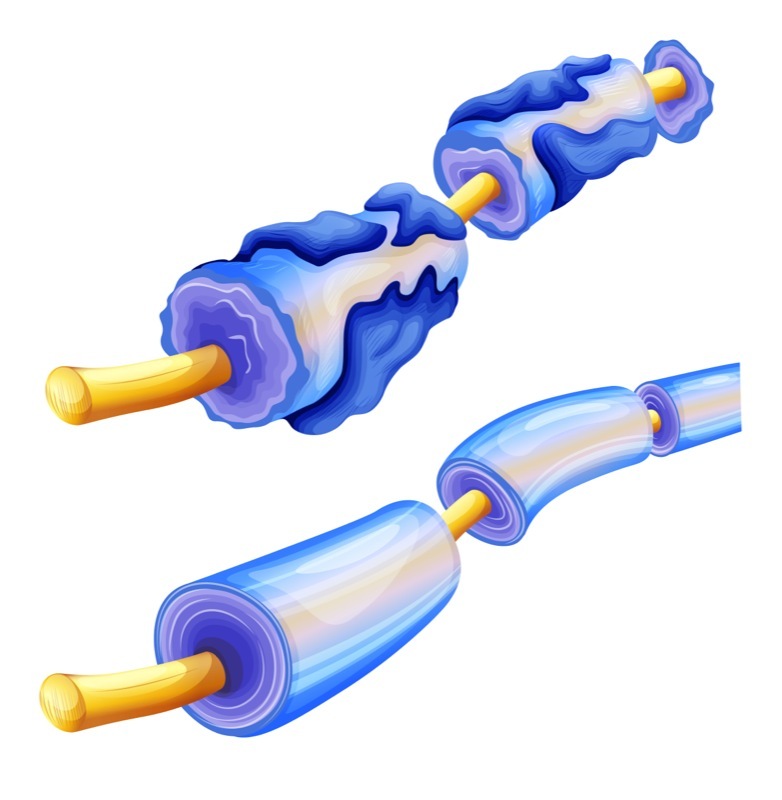
Nerve impulses travel in a “dual cable” with myelin, playing additional roles to what was previously thought, new research has found. This discovery advances human knowledge of how brain connections work, and may help scientists understand more accurately what happens when myelin is lost — which is what occurs in diseases like multiple sclerosis (MS).
The study reporting the findings, titled “Saltatory Conduction along Myelinated Axons Involves a Periaxonal Nanocircuit,” was published in the journal Cell.
Nerve cell fibers, or axons, of vertebrates — animals with backbones, including humans — are covered by compact layers of a lipid-rich (fatty) substance known as myelin.
Myelin serves as a kind of electrical insulator that makes nerve impulses travel fast, so as to maintain high-speed communication between nerve cells, across the peripheral and central nervous systems (brain and spinal cord). In most densely myelinated axons, the conduction velocity can reach 70–120 meters per second, the speed of a race car.
At the basis of this rapid conduction are myelin-free gaps, called nodes of Ranvier, placed along the axon.
Nerve impulses, known as action potentials, can propagate quickly along the axon because they “jump” from one node of Ranvier to the next, a process known as saltatory conduction. An impulse jumps from node to node down the full length of an axon, speeding its arrival and transmission to the next nerve cell, compared with action potentials that travel along unmyelinated axons.
Scientists have known about this process for many decades. But they had been missing, until now, one piece of the detailed picture of how these electrical circuits take place, and what happens when myelin is damaged, such as in demyelinating diseases like MS.
While the view that myelin is an insulator with minimal or no electrical activity had been widely accepted, some scientists have proposed alternative models in which impulses can actually travel inside myelin or just below it.
Now, a team led by researchers at the Netherlands Institute for Neuroscience (NIN) sought to further assess signal transmission in myelinated neurons. They used a new technique that makes electrical currents visible, called high-speed optical recordings, and combined it with computational modeling to determine the specific properties of myelin sheaths in rat neurons.
The team also used a high-resolution microscopy technique, called electron microscopy, to measure the distance between the nerve cell membrane — the border that separates the nerve cell from the external environment — and the myelin sheath.
The evidence showed that the axon and the myelin sheath surrounding it are separated, creating a second conduction pathway that runs just below the myelin sheaths and above the nerve cell membrane, known as the submyelin space.
The distance between the nerve cell and myelin sheath turned out to be 12 nanometers, which corresponds to a size 10,000 times thinner than a human hair.
Such observations match a proposed model for the transmission of nerve impulses referred to as the “double cable.”
“All the findings together showed that instead of being an insulating sheath, myelin creates an additional layer like coaxial cables producing multiple waves of electrical potentials traveling in a more complicated manner than was envisioned earlier,” Maarten Kole, PhD, group leader at NIN and the study’s senior author, said in a press release.
According to the team, the findings open new avenues to understand how brains warrant the rapid spread of impulses, and how damage to the myelin sheath and submyelin spaces “may cause the conduction impairments observed in demyelinating diseases.”
The findings also allow researchers to fine-tune their models, and create tools to better understand such diseases.
In patients with MS, in particular, myelin loss leads to a decline in strength, balance, and coordination, limiting a person’s mobility. The team believes that, to better treat and prevent MS, it is important to know exactly how myelin works — and to predict what happens if it stops working.
“Our work now may provide reliable predictions of how impulses travel along the highways without myelin,” said Kole, also a professor at Utrech University, in the Netherlands.
“This finding contributes to the understanding of the cellular changes occurring in MS,” he said.
The research project was funded by the European Research Council (ERC), the National Multiple Sclerosis Society, and the Netherlands Organization for Scientific Research.